- 1Center for Biomedical Research (CIBM), CIBER-EHD, ibs.Granada, Department of Pharmacology, University of Granada, Granada, Spain
- 2Stromal Cells and Immunology Group, Pfizer, University of Granada, Andalusian Regional Government Centre of Genomics and Oncological Research (GENYO), Granada, Spain
Inflammatory bowel disease (IBD) is a group of chronic inflammatory conditions of the gastrointestinal tract characterized by an exacerbated mucosal immune response. Macrophages play pivotal roles in the maintenance of gut homeostasis but they are also implicated in the pathogenesis of IBD. They are highly plastic cells and their activation state depends on the local environment. In the healthy intestine, resident macrophages display an M2 phenotype characterized by inflammatory energy, while inflammatory M1 macrophages dominate in the inflamed intestinal mucosa. In this regard, modifying the balance of macrophage populations into an M2 phenotype has emerged as a new therapeutic approach in IBD. Multipotent mesenchymal stromal cells (MSCs) have been proposed as a promising cell-therapy for the treatment of IBD, considering their immunomodulatory and tissue regenerative potential. Numerous preclinical studies have shown that MSCs can induce immunomodulatory macrophages and have demonstrated that their therapeutic efficacy in experimental colitis is mediated by macrophages with an M2-like phenotype. However, some issues have not been clarified yet, including the importance of MSC homing to the inflamed colon and/or lymphoid organs, their optimal route of administration or whether they are effective as living or dead cells. In contrast, the mechanisms behind the effect of MSCs in human IBD are not known and more data are needed regarding the effect of MSCs on macrophage polarization that would support the observation reported in the experimental models. Nevertheless, MSCs have emerged as a novel method to treat IBD that has already been proven safe and with clinical benefits that could be administered in combination with the currently used pharmacological treatments.
Functional Plasticity of Macrophages
Macrophages are tissue resident phagocytic cells that play fundamental roles in steady-state tissue homeostasis, regulation of the inflammatory response and host defense. Macrophages respond promptly to environmental stimuli using multiple receptors that results in a specific and optimized activation state ready to deal with the task at hand (Murray, 2017).
The activation states of macrophages were initially divided into classically activated M1 macrophages [induced by interferon (IFN)-γ], which participate in the anti-microbial response, and alternatively activated M2 macrophages [induced by interleukin (IL)-4], which protect against parasites and participate in wound healing/tissue remodeling (Stein et al., 1992; Hill Charles et al., 2000). While the prototypic M1/M2 polarization states are clearly established in vitro, their distinction in vivo has been difficult due to the multitude of stimuli resulting in mixed M1/M2 macrophage activation states (Martinez and Gordon, 2014). Recent data points to a continuum of activation states where stimulation of macrophages with lipopolysaccharide (LPS), tumor necrosis factor (TNF)-α, IL-10, IL-13, transforming growth factor (TGF)-β, glucocorticoids (GC), or immune complexes (IC) gives rise to similar but distinct transcriptional and functional macrophage activation states along the M1-M2 axis (Martinez and Gordon, 2014; Murray et al., 2014; Xue et al., 2014; Murray, 2017). In addition, stimulation of macrophages with free fatty acids, high-density lipoprotein (HDL) or with stimuli involved in chronic inflammation [including prostaglandin (PG) E2 and the toll like receptor (TLR) 2 ligand P3C] results in macrophage activation states that go outside the M1-M2 continuum (Popov et al., 2008; Xue et al., 2014) showing the complexity of macrophage activation and function (Figure 1).
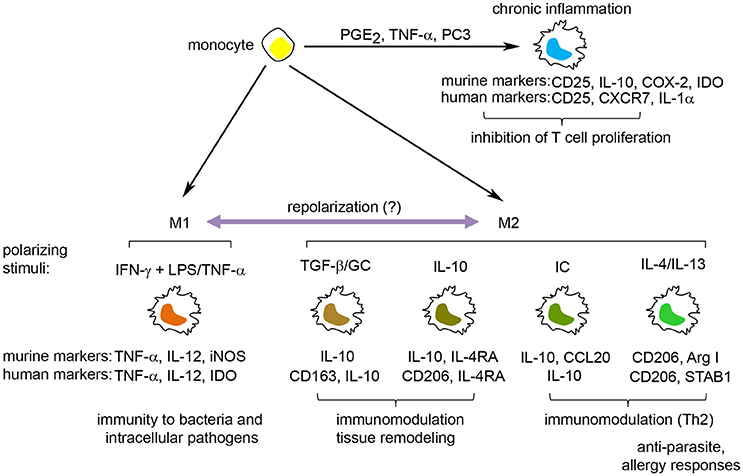
Figure 1. The spectrum of macrophage activation. Macrophages can respond to a wide range of stimuli, resulting in the induction of a spectrum of macrophage activation states. These include M1 macrophages, involved in the protection against bacteria, and M2 macrophages, induced by Th2 cytokines, anti-inflammatory cytokines (IL-10, TGF-β), immune complexes and glucocorticoids, and participate in anti-parasite immune responses, tissue remodeling/wound healing and inhibition of immune responses. Furthermore, stimuli associated with chronic inflammation, including PGE2, TNF-α and the TLR2-ligand PC3, induce a macrophage activation state distinct from the M1/M2 macrophages that have the potential to inhibit T cell proliferation. Defining molecules for murine and human M1 and M2 macrophages are indicated under each specific polarization state. GC, glucocorticoids; IC, immune complexes; IDO, indoleamine 2,3-dioxygenase; iNOS, inducible nitric oxide synthase.
A large number of surface molecules, cytokines, intracellular enzymes, and transcription factors are used to identify and differentiate between discrete macrophage activation states. M1 macrophages are generally distinguished by their high production of proinflammatory cytokines (IL-6, IL-12, TNF-α) and the expression of inducible nitric oxide synthase (iNOS) (in mouse) and indolamine 2,3,-dioxygenase (IDO) (in human). Markers for M2 macrophages encompass both stimuli-specific molecules (Xue et al., 2014) and more general M2 markers, such as CD206 (mannose receptor) and arginase I (Murray et al., 2014). CD206 is a surface marker for murine (Stein et al., 1992) and human (Murray et al., 2014) M2 macrophages induced by IL-4/IL-13 or IL-10 (Mantovani et al., 2004). In contrast, arginase I expression and activity are frequently used as a marker for murine, but not human, M2-polarized macrophages (Thomas and Mattila, 2014). Finally, IL-10 is one of the most used markers for M2 macrophages due to its higher expression in several M2 macrophage polarization states (except for IL-4/IL-13-induced M2 macrophages) compared to M1 macrophages.
As mentioned above, macrophages are functionally plastic cells whose activation states are dictated by the relative concentration of M1/M2 polarizing stimuli in the local environment (Wynn et al., 2013; Smith et al., 2016). As a consequence, switches between macrophage polarization states (M1 to M2 and vice versa) can be seen during responses to infection, wound healing and disease, including cancer (Qian and Pollard, 2010; Wynn et al., 2013). However, it is not clear whether these changes in macrophage activation status are due to (i) recruitment of new monocytes and their subsequent activation in response to changed local cues or (ii) repolarization of M1 macrophages into M2 macrophages or vice versa, or (iii) a combination of both (Italiani and Boraschi, 2014). While the repolarization of M1 into M2 macrophages has been described (Porcheray et al., 2005; Davis et al., 2013; Tarique et al., 2015; Kudlik et al., 2016), a recent study showed that human and murine M1 macrophages failed to convert into M2 cells upon IL-4 exposure in vitro and in vivo due to mitochondrial dysfunction (Van Den Bossche et al., 2016).
Role of Macrophages in IBD
Inflammatory bowel disease (IBD) is a group of chronic gastrointestinal inflammatory diseases that include Crohn's disease (CD) and ulcerative colitis (UC). They both feature alternating periods of remissions and relapses, characterized by uncontrolled intestinal inflammation, with disabling symptoms like diarrhea, abdominal pain, fever, clinical signs of bowel obstruction, as well as passage of blood or mucus or both. This implies extended medical and/or surgical procedures that impair the patients' quality of life. At present, the etiology of IBD is not fully elucidated, and most probably results from an intricate combination of four major factors: genetic predisposition, compositional and metabolic changes in the intestinal microbiota (dysbiosis), environmental exposures, and deregulation of mucosal immune responses (de Souza et al., 2017).
There is a consensus that IBD appears in genetically susceptible individuals who display an altered intestinal barrier function with increased paracellular permeability. These patients develop an exaggerated immune response toward the intestinal microbiota that triggers the chronic intestinal inflammation. In this scenario, cells from both the innate [including intestinal epithelial cells, monocytes/macrophages, neutrophils, and dendritic cells (DCs)] and adaptive (including T- and B-cells) arms of the mucosal immune system and their secreted mediators (cytokines, chemokines, eicosanoids and reactive oxygen and nitrogen species) are involved in the pathogenesis of IBD (Xavier and Podolsky, 2007).
The largest population of macrophages in the body resides in the gastrointestinal mucosa (Lee et al., 1985), where they play pivotal roles in the maintenance of epithelial and immunological homeostasis (Pull et al., 2005; Isidro and Appleyard, 2016). The intestinal macrophage pool is continuously replenished from Ly6Chigh monocyte precursors recruited in a CCR2-dependent manner into the intestinal lamina propria (Bain et al., 2014). In steady state, the local microenvironment of the intestinal mucosa induces monocytic precursors to acquire the homeostatic phenotypic properties of intestinal-resident macrophages (M2 phenotype). These intestinal macrophages display scavenger and bacteriocidal activities together with an inflammatory anergy which is, in part, induced by commensal microbiota (Ueda et al., 2010; Zigmond et al., 2014) and intestinal stromal cell cues (Smythies et al., 2005; Maheshwari et al., 2011). They are also characterized by an anti-inflammatory gene expression profile that involves the up-regulation of IL-10, a cytokine with anti-inflammatory properties (Bain and Mowat, 2014). Importantly, IL-10 plays a key role in regulating the pro-inflammatory responses of murine and human intestinal macrophages and mutations in its receptor, IL-10R, result in acute IBD in humans (Glocker et al., 2009) and severe spontaneous colitis in mice (Zigmond et al., 2014). However, in human IBD and murine experimental colitis, the CD14+ monocytes that are recruited into the inflamed colonic mucosa fail to become anergic. Instead, they turn into inflammatory macrophages (M1 phenotype) that produce high levels of proinflammatory cytokines (including IL-1β, TNF-α, IL-23), nitric oxide and reactive oxygen intermediates (Grimm et al., 1995; Tokuyama et al., 2005; Joeris et al., 2017). Proinflammatory CD14+ macrophages also home to the mesenteric lymph nodes (MLN) where they promote disease (Li et al., 2017). In this regard, the inflammatory macrophages outnumber the resident population, and all the secreted pro-inflammatory mediators have a deleterious impact on epithelial permeability, increasing pathogen invasion (Du Plessis et al., 2013) and promoting accumulation of IL-17-producing innate and adaptive leukocytes (Coccia et al., 2012).
Modulation of Macrophage Activation as a Treatment for IBD
Considering all the above, the pharmacological alteration of the balance of macrophage populations in the inflamed intestine, especially promoting an increase in the anti-inflammatory M2 phenotype, is becoming an attractive therapeutic approach in IBD. Firstly, administration of in vitro generated M2 macrophages, secreting high levels of IL-10, has been found to lessen the severity of colitis in mice (Hunter et al., 2010; Anderson et al., 2013b; Leung et al., 2013). Secondly, mice infected with schistosome worms acquired a macrophage-dependent protection against DSS-induced colitis. This protection was not associated with any known M2 macrophage activation state or IL-10/TGF-β expression. However, transfer of colon lamina propria macrophages from infected mice significantly suppressed colitis in recipient mice (Smith et al., 2007), highlighting the multitude of immunomodulatory macrophage activation states. Thirdly, patients with active CD showed fewer CD68+CD206+ macrophages in the inflamed mucosa (which indicates alternatively activated macrophages) than patients with inactive CD (Hunter et al., 2010). Interestingly, the anti-TNF-α monoclonal antibody, infliximab, which is successfully used in the treatment of human IBD (Danese et al., 2015), was found to induce regulatory macrophages (CD68+CD206+) in vitro (Vos et al., 2011) and in patients with IBD responding to treatment (Vos et al., 2012).
Multipotent Mesenchymal Stromal Cells
Multipotent mesenchymal stromal cells (MSCs) are non-hematopoietic, perivascular cells with tissue regenerative and immunomodulatory abilities that have emerged as a promising cell-therapy for regenerative medicine, autoimmune disease and cancer. The interest in MSCs began when Friedenstein originally described the existence of a rare non-hematopoietic bona fide stem cell in the bone marrow that could give rise to multiple skeletal tissues (bone, cartilage, and fibrous tissue) when transplanted in vivo (reviewed in Friedenstein, 1990). These cells were designated osteogenic stem cells or bone marrow (BM) stromal stem cells and were later found to promote bone remodeling and hematopoiesis in vivo (Bianco et al., 2008; Méndez-Ferrer et al., 2010). Subsequently, cells with similar morphology and in vitro differentiation potential were isolated by plastic adherence from several adult and neonatal tissues and organs, including adipose tissue, muscle, umbilical cord and placenta (da Silva Meirelles, 2006). These cells were named “multipotent mesenchymal stromal cells,” to distinguish them from the osteogenic stem cells/BM stromal stem cells described by Friedenstein (Owen and Friedenstein, 1988). The current minimal criteria to define human MSCs are (i) plastic adherence under normal culture conditions in vitro (ii) expression of CD73, CD90 and CD105 and lack of expression of CD45, CD34, CD14 or CD11b, CD79α or CD19 and HLA-DR surface molecules and (iii) differentiation into osteoblasts, adipocytes and chondroblasts in vitro (Dominici et al., 2006). Although the in vivo function and differentiation potential of MSCs appear to depend on their tissue of origin (Sacchetti et al., 2016), in vitro expanded MSCs can migrate to sites of injury/inflammation (Kidd et al., 2009), secrete trophic factors and are potent regulators of the innate and adaptive immune responses in vitro and in vivo (Di Nicola et al., 2002; Constantin et al., 2009; Gonzalez-Rey et al., 2009; Anderson et al., 2013a, 2017; Gao et al., 2016) (Figure 2). Several clinical trials have evaluated the immunomodulatory and tissue regenerative potential of both autologous and allogeneic MSCs with promising results (Quarto et al., 2001; Connick et al., 2012; von Bahr et al., 2012).
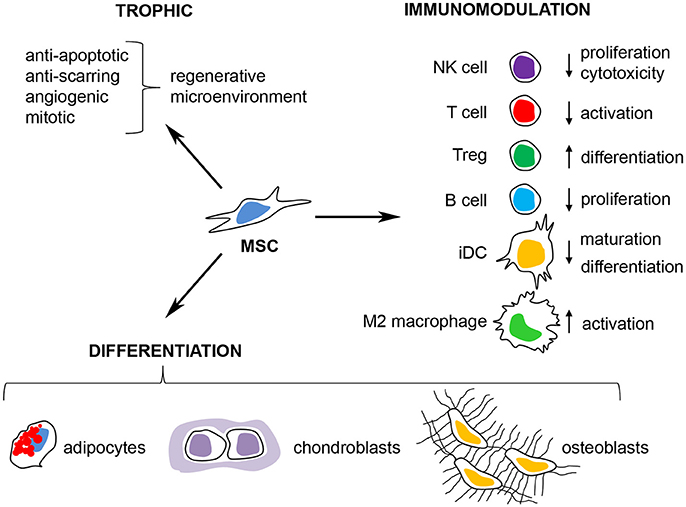
Figure 2. Therapeutic properties of multipotent mesenchymal stromal cells. MSCs have emerged as a promising cell therapy for inflammatory/autoimmune diseases and in regenerative medicine due to their (i) secretion of trophic factors that promote a regenerative microenvironment, (ii) their capacity to differentiate into adipocytes, osteoblasts, and chondroblasts in vitro and in vivo and (iii) their immunomodulatory capacity where MSCs can inhibit the activation of T cells, NK cells, and B cells, prevent the maturation of dendritic cells (iDC) and promote immunological tolerance through the induction of M2 macrophages and regulatory T cells (Tregs).
MSCs and Their Potential Application as a Cell Therapy for IBD
MSCs are nowadays considered a promising future treatment of IBD. Administration of syngeneic, allogeneic and xenogeneic MSCs ameliorates experimental colitis by regulating Th1/Th17 responses, inducing regulatory T cells (Tregs) and reducing the levels of proinflammatory cytokines in the inflamed colon (Hayashi et al., 2008; Gonzalez-Rey et al., 2009; Li et al., 2013). Clinical trials using MSCs for the treatment of fistulizing CD (Ciccocioppo et al., 2011; Panés et al., 2016; Dietz et al., 2017), luminal CD (Duijvestein et al., 2010; Forbes et al., 2014; Zhang et al., 2017) and UC (Hu et al., 2016) have shown encouraging clinical responses and safety.
Effect of MSCs on Macrophage Polarization in Vitro
Numerous studies have demonstrated that MSCs can induce immunomodulatory M2-like macrophages in vitro that can inhibit T cell and NK cell function and induce Tregs (Gonzalez-Rey et al., 2009; Anderson et al., 2013b; Melief et al., 2013a; Chiossone et al., 2016). Conditioned medium of murine adipose tissue-derived MSCs (ASCs) was found to induce regulatory macrophages in vitro, which were distinct from IL-4 activated macrophages. These ASC-induced macrophages were characterized by high arginase I activity, IL-10 production and expression of LIGHT, heme oxygenase (HO)-1 and arginase II, possessing immunomodulatory capacity in vitro and in experimental colitis and sepsis (Anderson et al., 2013b). Furthermore, murine BM-MSCs have been shown to induce high IL-10 production in both M-CSF-derived BM-macrophages and in thioglycollate-induced peritoneal macrophages (Cho et al., 2014; Kudlik et al., 2016). Importantly, some studies have suggested that MSCs can repolarize M1 macrophages (induced by M-CSF + LPS or GM-CSF + IFN-γ) into IL-10 expressing M2 macrophages (Németh et al., 2009; Manferdini et al., 2017). In general, the main MSC-derived molecule that promotes the M2 activation state is PGE2, although other effector molecules could also be involved (Table 1).
Similarly, human ASCs (Manferdini et al., 2017), BM-MSCs (Melief et al., 2013a; Chiossone et al., 2016; Vasandan et al., 2016), and amniotic MSCs (Magatti et al., 2017) induced an M2 activation state in CD14+ monocytes (stimulated or not with either M-CSF or GM-CSF). In all cases, the M2 activation state was characterized by CD206 expression and IL-10 secretion and when analyzed, depended on MSC-derived PGE2 (Table 1). One study also implicated IDO activity in MSCs in the induction of CD206+IL-10high M2 macrophages (François et al., 2012). However, IDO is also expressed in some types of M2-like macrophages that could make the effects of pharmacological inhibition of IDO on MSC-mediated macrophage polarization difficult to interpret (Xue et al., 2014; Selleri et al., 2016).
In addition, exosomes from murine and human MSCs have been shown to induce IL-10highCD206+ macrophages (Henao Agudelo et al., 2017; Lo Sicco et al., 2017; Mao et al., 2017a). Song et al. found that human umbilical cord (UC)-MSC-derived exosomes transferred microRNA (miR)-146a to macrophages, inducing an M2 phenotype (Song Y. et al., 2017). Interestingly, Phinney et al. showed that MSCs exposed to oxidative stress shed exosomes containing depolarized mitochondria, which are taken up by macrophages. However, in order to prevent an TLR-mediated inflammatory response to the engulfed mitochondria, MSCs simultaneously shed miR-containing exosomes that repress TLR signaling and production of inflammatory mediators in macrophages (Phinney et al., 2015).
MSCs can also prevent the GM-CSF/IL-4-induced differentiation of monocytes into immature dendritic cells (iDCs) in vitro. Here, the presence of MSCs promotes a CD14+CD1a−IL-10high “M2-like” macrophage activation state with low allostimulatory capacity. To date, several MSC-derived mediators have been implicated in this process, including hepatocyte growth factor (HGF) (Deng et al., 2016), IL-6 (Melief et al., 2013a), and lactate (Selleri et al., 2016).
In summary, MSCs can induce M2 polarization of monocytes, primed M1 macrophages (by GM-CSF or thioglycollate) and polarized M1 macrophages (by LPS and/or IFN-γ). MSCs also promote an M2-like macrophage activation state when in contact with monocytes under dendritic cell stimuli (GM-CSF/IL-4) and M1 stimuli (LPS + IFN-γ) (Figure 3).
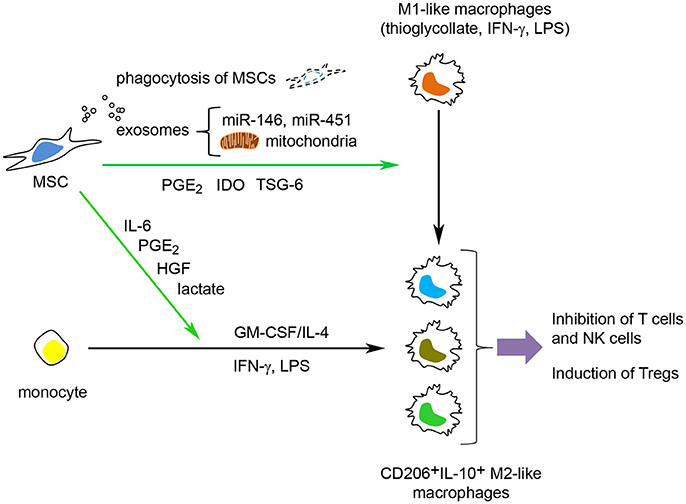
Figure 3. MSCs can modulate macrophage function through several mechanisms. MSCs can induce M2 polarization of monocytes, primed M1 macrophages (stimulated with GM-CSF or thioglycollate) and polarized M1 macrophages (polarized with LPS and/or IFN-γ). MSCs can also inhibit the GM-CSF/IL-4-mediated differentiation of monocytes into immature dendritic cells and prevent the LPS/IFN-γ-mediated polarization into M1 macrophages and instead promote a M2-like macrophage activation state. MSCs use both active (TSG-6, PGE2, cytokines/growth factors and lactate) and passive (phagocytosis of MSCs by macrophages, transfer of microRNAs and mitochondria to macrophages) mechanisms to modulate macrophage function.
Effects of MSCs on Macrophage Polarization in Vivo
MSCs have been revealed to modulate macrophage polarization in models of inflammatory/autoimmune diseases and tissue regeneration (Carty et al., 2017), including sepsis (Németh et al., 2009) wound healing (Zhang et al., 2010) and spinal cord injury (Nakajima et al., 2012). Several studies have linked the therapeutic efficacy of MSCs in experimental colitis to the induction/increase of macrophages with an M2-like phenotype (Liu et al., 2015; Markovic et al., 2015; Mao et al., 2017b; de Aguiar et al., 2018). Song W. J. et al. (2017), showed that human ASCs, through their secretion of tumor necrosis factor-stimulated gene (TSG)-6, induced M2 macrophage polarization in the colon and reduced disease severity in DSS-induced colitic mice. Parekkadan et al. reported that intravenous injection of BM-MSCs induced CD4+foxp3+ Tregs and prevented TNBS-induced colitis. Chemical (clodronate treatment) or surgical depletion (splenectomy) of splenic CD11b+ cells abolished the therapeutic effect of the MSCs. Moreover, the injection of CD11b+ macrophages co-cultured with MSCs, but not skin fibroblasts, also reduced colitis (Parekkadan et al., 2011).
Supporting the above, several reports have demonstrated that MSCs injections can inhibit the infiltration of macrophages into the inflamed colon. de Aguiar et al. described that the administration of murine ASCs into mice during the induction of DSS colitis reduced the infiltration of CD11b+F4/80+ macrophages into the MLNs and colonic lamina propria. Also, MSC-injection increased the protein levels of arginase-1 in the colon, suggestive of a M2 macrophage induction (de Aguiar et al., 2018). Similarly, the infiltration of monocytes/macrophages into the inflamed colon was significantly decreased in human amnion-MSC-treated rats (Onishi et al., 2015) and in human UC-MSC-treated DSS-colitic mice (Mao et al., 2017b).
Is the Homing and Viability of MSCs Important for their Therapeutic Effects in Colitis/IBD?
Injected MSCs have been shown to home to both the inflamed/injured colon and/or the MLNs and spleens in colitic mice (Gonzalez-Rey et al., 2009; Liang et al., 2011; Parekkadan et al., 2011; Castelo-Branco et al., 2012; Fan et al., 2012; Mao et al., 2017b; Takeyama et al., 2017). However, some recent studies have reported that only a minor fraction (0.001–1%) of the injected MSCs actually reaches the inflamed colon. The lack of homing in different studies can be due to inadequate culture conditions (De Becker and Van Riet, 2016), prolonged trypsinization (Chamberlain et al., 2008) or high passage number of the MSCs, since they lose the expression of chemokine receptors upon in vitro culturing (Honczarenko et al., 2006). Activation of MSCs using inflammatory cytokines, like IFN-γ, TNF-α, and IL-1β, has been demonstrated to induce their surface expression of chemokine receptors and integrins (Duijvestein et al., 2011b; De Becker and Van Riet, 2016). Preactivation of MSCs with IL-1β upregulated the expression of CXCR4 and increased their homing to the spleen, MLNs and the inflamed colon of DSS-treated mice (Fan et al., 2012). Duijvestein et al. also showed that pretreatment with IFN-γ increased the homing of MSCs to the inflamed intestine and potentiated their therapeutic effect in both DSS- and TNBS-induced colitis (Duijvestein et al., 2011b).
However, several recent reports suggest that the migration of MSCs to the inflamed colon and/or lymphoid organs is not necessary for their therapeutic effect in experimental colitis. Instead, intraperitoneally injected MSCs can remain trapped in the peritoneal cavity, causing a suppression of the intestinal inflammation (Bazhanov et al., 2016; Song Y. et al., 2017). In this line, Sala et al. found that intraperitoneal administration of MSCs reduced DSS-induced colitis via production of TSG-6, independently of their homing to the intestine. In fact, the injected MSCs formed aggregates in the peritoneal cavity, which remained external to the bowel wall vessels, but able to promote the induction of colonic macrophages with a regulatory phenotype (CD11b+F4/80+IL-10highiNOSlow) (Sala et al., 2015).
As discussed above, the therapeutic potential of MSCs in intestinal inflammation has been linked to their capacity to actively modulate the immune system, either in lymphoid organs and the inflamed colon or through immunomodulation in the peritoneal cavity. However, it is far from clear whether MSCs are effective in vivo as living or dead/dying cells (Bianco et al., 2013; Sacchetti et al., 2016), and some recent studies propose that MSCs can modulate macrophage function through passive mechanisms. Luk et al. showed that heat-inactivated MSCs could not inhibit T cell and B cell activation/proliferation but could modulate monocyte function in response to LPS (Luk et al., 2016). In another study, Braza et al. found that intravenously injected MSCs were phagocytosed by murine lung macrophages that acquired a IL-10highTGF-βhighIL-6low phenotype in comparison to macrophages that had not ingested MSCs (Zigmond et al., 2014; Braza et al., 2016). In the same line, Song et al. described that intraperitoneal injections of freeze-thaw extracts from human UC-MSCs induced M2 polarization of intraperitoneal macrophages and reduced DSS-colitis (Song J. et al., 2017). In summary, MSCs can induce M2 macrophages in vivo through active or passive mechanisms, regardless of their homing to the inflamed colon and secondary lymphoid organs.
In contrast to the plethora of information on how MSCs modulate the immune system in murine colitis, the mechanisms behind their effect in human IBD are not known. Due to technical difficulties, only a few clinical studies have included sample collection in order to evaluate inflammation and immune responses in IBD patients receiving either local or systemic injections of MSCs. Hu et al. showed that administration of human UC-MSCs reduced the histological score in patients with UC, as evidenced by the improvement of the mucosal surface, mucin content in goblet cells, crypt abscesses, gland collapse, and reduction of the inflammatory infiltrate (Hu et al., 2016). Also, a decreased amount of pro-inflammatory cytokines (TNF-α, IL-1β) was observed in mucosal biopsies in patients with refractory luminal CD receiving intravenous infusions of autologous BM-MSCs (Duijvestein et al., 2010). Taken together, although the beneficial effects of MSCs have been reported in human IBD, data is lacking on the effect of MSCs on macrophage polarization that support the observations reported in preclinical studies in experimental models of rodent colitis.
Advantages and Disadvantages using MSCs for IBD
Current treatments for IBD include aminosalicylates, corticosteroids, immune-suppressants, antibiotics, and biological drugs (Bernstein, 2015). Despite their efficacy in patients with either UC or CD, their chronic use is frequently associated with severe side effects, including osteoporosis/osteonecrosis, infectious complications and development of lymphoma among others (Rutgeerts et al., 2005; Siegel, 2011; Garg et al., 2014). For this reason, there is a real need for the development of new treatments combining efficacy and safety, which could be the case of MSCs.
The main advantages of using MSCs for the treatment of IBD are: (i) administration of MSCs is safe with clinical benefits for the treatment of IBD, especially fistulizing CD. (ii) MSCs are easily isolated and can be efficiently cultured in vitro. (iii) MSC function is not altered by the pharmacological treatments used in IBD (azathioprine, methotrexate, 6-mercaptopurine and biologicals like anti-TNF-α), thus supporting their use in combination with these drugs (Duijvestein et al., 2010, 2011a). (iv) MSCs could be an option for CD patients refractory to current drug treatments. Interestingly, it has been shown that MSCs-treated patients can also regain responsiveness to those drugs (Ciccocioppo et al., 2015). (v) MSCs can migrate to the inflamed colon and lymphoid organs. Some studies have reported low homing efficiency probably due to the entrapment of MSCs in lungs and loss of homing receptors during in vitro expansion. Pretreatment of MSCs with inflammatory cytokines improves their homing to the inflamed intestine and enhances their therapeutic efficacy in experimental colitis. However, migration to the colon might not be necessary for their therapeutic effect.
Nevertheless, there are some aspects that need to be solved in order to definitively establish the therapeutic use of MSCs: (i) It is not clear whether autologous MSCs from patients with IBD possess the same therapeutic capacity as MSCs from healthy donors (Chinnadurai et al., 2015; Serena et al., 2017). A solution could be the use of allogeneic MSCs, which are considered immune evasive due to their low or absent expression of MHC class I and II and their immunosuppressive nature (Ankrum et al., 2014; Molendijk et al., 2015; Panés et al., 2016). However, IFN-γ can increase the expression of both MHC class I and II on MSCs (Romieu-Mourez et al., 2007) that would make allogeneic MSCs more susceptible to rejection in an immune-competent host. This could be a problem for applications where long term engraftment of MSCs is necessary for a clinical effect. (ii) Although MSCs administration can increase the pool of Tregs, some long term follow up studies (>6 months after intervention) suggest that the beneficial effects of the MSC-therapy wear off with time (Dave et al., 2017). This indicates a failure of establishing immunological tolerance and suggests the necessity of repeated treatments. (iii) No transformation of MSCs has been detected in any of the patients that have received MSCs injections in clinical trials. However, due to the reported pro-tumorigenic effect of MSCs in vitro and in vivo (Wei et al., 2015; Chen et al., 2017), further studies need to address whether infusion of MSCs increases the rate of colorectal cancer in IBD patients that already have an increased risk (Ullman and Itzkowitz, 2011). (iv) Moreover, since MSCs promote intestinal immunotolerance, there is a risk of infection after the cell administration. This could be addressed by the use of anti-microbial drugs (Wei et al., 2017).
In summary, MSCs can be considered as an attractive therapeutic strategy for the efficient and safe management of human IBD. Although the exact mechanisms involved require further clarification, the impact of macrophage polarization toward the anti-inflammatory M2 phenotype seems to be especially relevant.
Author Contributions
All authors listed have made a substantial, direct and intellectual contribution to the work, and approved it for publication.
Conflict of Interest Statement
The authors declare that the research was conducted in the absence of any commercial or financial relationships that could be construed as a potential conflict of interest.
Acknowledgments
This work was supported by Junta de Andalucia (Spain) (research grants CTS 164 and PI-0206-2016), the Spanish Ministry of Economy and Competitiveness (research grant AGL2015-67995-C3-3-R) and Fondo de Investigaciones Sanitarias ISCIII (Spain) (research grant PI15/00794) cofinanced by the Fondo Europeo de Desarollo Regional (FEDER) from the European Union. LH-G is a Ph.D. student from the Postgraduate Program Medicina Clinica y Salud Publica at the University of Granada (Spain) (FPU16/05651), supported by Initiation-Research fellowship from the Vice-Rectorate of Scientific Policy and Research of University of Granada. PA has a Miguel Servet Type II Contract (CPII15/00032), cofinanced by the Fondo Europeo de Desarollo Regional (FEDER) from the European Union.
References
Anderson, P., Carrillo-Gálvez, A. B., García-Pérez, A., Cobo, M., Martín, F., and Menendez, P. (2013a). CD105 (Endoglin)-negative murine mesenchymal stromal cells define a new multipotent subpopulation with distinct differentiation and immunomodulatory capacities. PLoS ONE 8:e76979. doi: 10.1371/journal.pone.0076979
Anderson, P., Gonzalez-Rey, E., O'valle, F., Martin, F., Oliver, F. J., and Delgado, M. (2017). Allogeneic adipose-derived mesenchymal stromal cells ameliorate experimental autoimmune encephalomyelitis by regulating self-reactive T cell responses and dendritic cell function. Stem Cells Int. 2017:2389753. doi: 10.1155/2017/2389753
Anderson, P., Souza-Moreira, L., Morell, M., Caro, M., O'valle, F., Gonzalez-Rey, E., et al. (2013b). Adipose-derived mesenchymal stromal cells induce immunomodulatory macrophages which protect from experimental colitis and sepsis. Gut 62, 1131–1141. doi: 10.1136/gutjnl-2012-302152
Ankrum, J. A., Ong, J. F., and Karp, J. M. (2014). Mesenchymal stem cells: immune evasive, not immune privileged. Nat. Biotechnol. 32, 252–260. doi: 10.1038/nbt.2816
Bain, C. C., and Mowat, A. M. (2014). Macrophages in intestinal homeostasis and inflammation. Immunol. Rev. 260, 102–117. doi: 10.1111/imr.12192
Bain, C. C., Bravo-Blas, A., Scott, C. L., Gomez Perdiguero, E., Geissmann, F., Henri, S., et al. (2014). Constant replenishment from circulating monocytes maintains the macrophage pool in the intestine of adult mice. Nat. Immunol. 15, 929–937. doi: 10.1038/ni.2967
Bazhanov, N., Ylostalo, J. H., Bartosh, T. J., Tiblow, A., Mohammadipoor, A., Foskett, A., et al. (2016). Intraperitoneally infused human mesenchymal stem cells form aggregates with mouse immune cells and attach to peritoneal organs. Stem Cell Res. Ther. 7:27. doi: 10.1186/s13287-016-0284-5
Bernstein, C. N. (2015). Treatment of IBD: where we are and where we are going. Am. J. Gastroenterol. 110, 114–126. doi: 10.1038/ajg.2014.357
Bianco, P., Cao, X., Frenette, P. S., Mao, J. J., Robey, P. G., Simmons, P. J., et al. (2013). The meaning, the sense and the significance: translating the science of mesenchymal stem cells into medicine. Nat. Med. 19, 35–42. doi: 10.1038/nm.3028
Bianco, P., Robey, P. G., and Simmons, P. J. (2008). Mesenchymal stem cells: revisiting history, concepts, and assays. Cell Stem Cell. 2, 313–319. doi: 10.1016/j.stem.2008.03.002
Braza, F., Dirou, S., Forest, V., Sauzeau, V., Hassoun, D., Chesné, J., et al. (2016). Mesenchymal stem cells induce suppressive macrophages through phagocytosis in a mouse model of asthma. Stem Cells 34, 1836–1845. doi: 10.1002/stem.2344
Carty, F., Mahon, B. P., and English, K. (2017). The influence of macrophages on mesenchymal stromal cell therapy: passive or aggressive agents? Clin. Exp. Immunol. 188, 1–11. doi: 10.1111/cei.12929
Castelo-Branco, M. T., Soares, I. D., Lopes, D. V., Buongusto, F., Martinusso, C. A., do Rosario, A. Jr., et al. (2012). Intraperitoneal but not intravenous cryopreserved mesenchymal stromal cells home to the inflamed colon and ameliorate experimental colitis. PLoS ONE 7:e33360. doi: 10.1371/journal.pone.0033360
Chamberlain, G., Wright, K., Rot, A., Ashton, B., and Middleton, J. (2008). Murine mesenchymal stem cells exhibit a restricted repertoire of functional chemokine receptors: comparison with human. PLoS ONE 3:e2934. doi: 10.1371/journal.pone.0002934
Chen, K., Liu, Q., Tsang, L. L., Ye, Q., Chan, H. C., Sun, Y., et al. (2017). Human MSCs promotes colorectal cancer epithelial–mesenchymal transition and progression via CCL5/β-catenin/Slug pathway. Cell Death Dis. 8:e2819. doi: 10.1038/cddis.2017.138
Chinnadurai, R., Copland, I. B., Ng, S., Garcia, M., Prasad, M., Arafat, D., et al. (2015). Mesenchymal stromal cells derived from Crohn's patients deploy indoleamine 2,3-dioxygenase-mediated immune suppression, independent of autophagy. Mol. Ther. 23, 1248–1261. doi: 10.1038/mt.2015.67
Chiossone, L., Conte, R., Spaggiari, G. M., Serra, M., Romei, C., Bellora, F., et al. (2016). Mesenchymal stromal cells induce peculiar alternatively activated macrophages capable of dampening both innate and adaptive immune responses. Stem Cells 34, 1909–1921. doi: 10.1002/stem.2369
Cho, D.-I., Kim, M. R., Jeong, H.-Y., Chang Jeong, H., Jeong, M. H., Yoon, S. H., et al. (2014). Mesenchymal stem cells reciprocally regulate the M1/M2 balance in mouse bone marrow-derived macrophages. Exp. Mol. Med. 46:e70. doi: 10.1038/emm.2013.135
Ciccocioppo, R., Bernardo, M. E., Sgarella, A., Maccario, R., Avanzini, M. A., Ubezio, C., et al. (2011). Autologous bone marrow-derived mesenchymal stromal cells in the treatment of fistulising Crohn's disease. Gut 60, 788–798. doi: 10.1136/gut.2010.214841
Ciccocioppo, R., Gallia, A., Sgarella, A., Kruzliak, P., Gobbi, P. G., and Corazza, G. R. (2015). Long-term follow-up of Crohn disease fistulas after local injections of bone marrow-derived mesenchymal stem cells. Mayo Clin. Proc. 90, 747–755. doi: 10.1016/j.mayocp.2015.03.023
Coccia, M., Harrison, O. J., Schiering, C., Asquith, M. J., Becher, B., Powrie, F., et al. (2012). IL-1β mediates chronic intestinal inflammation by promoting the accumulation of IL-17A secreting innate lymphoid cells and CD4 + Th17 cells. J. Exp. Med. 209, 1595–1609. doi: 10.1084/jem.20111453
Connick, P., Kolappan, M., Crawley, C., Webber, D. J., Patani, R., Michell, A. W., et al. (2012). Autologous mesenchymal stem cells for the treatment of secondary progressive multiple sclerosis: an open-label phase 2a proof-of-concept study. Lancet Neurol. 11, 150–156. doi: 10.1016/S1474-4422(11)70305-2
Constantin, G., Marconi, S., Rossi, B., Angiari, S., Calderan, L., Anghileri, E., et al. (2009). Adipose-derived mesenchymal stem cells ameliorate chronic experimental autoimmune encephalomyelitis. Stem Cells 27, 2624–2635. doi: 10.1002/stem.194
da Silva Meirelles, L. (2006). Mesenchymal stem cells reside in virtually all post-natal organs and tissues. J. Cell Sci. 119, 2204–2213. doi: 10.1242/jcs.02932
Danese, S., Vuitton, L., and Peyrin-Biroulet, L. (2015). Biologic agents for IBD: practical insights. Nat. Rev. Gastroenterol. Hepatol. 12, 537–545. doi: 10.1038/nrgastro.2015.135
Dave, M., Jaiswal, P., and Cominelli, F. (2017). Mesenchymal stem/stromal cell therapy for inflammatory bowel disease: an updated review with maintenance of remission. Curr. Opin. Gastroenterol. 33, 59–68. doi: 10.1097/MOG.0000000000000327
Davis, M. J., Tsang, T. M., Qiu, Y., Dayrit, J. K., Freij, J. B., Huffnagle, G. B., et al. (2013). Macrophage M1/M2 polarization dynamically adapts to changes in cytokine microenvironments in Cryptococcus neoformans infection. MBio 4:e00264-13. doi: 10.1128/mBio.00264-13
de Aguiar, C. F., Castoldi, A., Andrade-Oliveira, V., Ignacio, A., da Cunha, F. F., Felizardo, R. J. F., et al. (2018). Mesenchymal stromal cells modulate gut inflammation in experimental colitis. Inflammopharmacology 26, 251–260. doi: 10.1007/s10787-017-0404-6
De Becker, A., and Van Riet, I. (2016). Homing and migration of mesenchymal stromal cells: how to improve the efficacy of cell therapy? World J. Stem Cells 8:73. doi: 10.4252/wjsc.v8.i3.73
de Souza, H. S. P., Fiocchi, C., and Iliopoulos, D. (2017). The IBD interactome: an integrated view of aetiology, pathogenesis and therapy. Nat. Rev. Gastroenterol. Hepatol. 14, 739–749. doi: 10.1038/nrgastro.2017.110
Deng, Y., Zhang, Y., Ye, L., Zhang, T., Cheng, J., Chen, G., et al. (2016). Umbilical cord-derived mesenchymal stem cells instruct monocytes towards an IL10- producing phenotype by secreting IL6 and HGF materials and methods. Sci. Rep. 6:37566. doi: 10.1038/srep37566
Di Nicola, M., Carlo-Stella, C., Magni, M., Milanesi, M., Longoni, P. D., Matteucci, P., et al. (2002). Human bone marrow stromal cells suppress T-lymphocyte proliferation induced by cellular or nonspecific mitogenic stimuli. Blood 99, 3338–3343. doi: 10.1182/blood.V99.10.3838
Dietz, A. B., Dozois, E. J., Fletcher, J. G., Butler, G. W., Radel, D., Lightner, A. L., et al. (2017). Autologous mesenchymal stem cells, applied in a bioabsorbable matrix, for treatment of perianal fistulas in patients with Crohn's disease. Gastroenterology 153, 59.e2–62.e2. doi: 10.1053/j.gastro.2017.04.001
Dominici, M., Le Blanc, K., Mueller, I., Slaper-Cortenbach, I., Marini, F., Krause, D., et al. (2006). Minimal criteria for defining multipotent mesenchymal stromal cells. The international society for cellular therapy position statement. Cytotherapy 8, 315–317. doi: 10.1080/14653240600855905
Du Plessis, J., Vanheel, H., Janssen, C. E. I., Roos, L., Slavik, T., Stivaktas, P. I., et al. (2013). Activated intestinal macrophages in patients with cirrhosis release NO and IL-6 that may disrupt intestinal barrier function. J. Hepatol. 58, 1125–1132. doi: 10.1016/j.jhep.2013.01.038
Duijvestein, M., Christine Vos, A. W., Roelofs, H., Wildenberg, M. E., Wendrich, B. B., Verspaget, H. W., et al. (2010). Autologous bone marrow-derived mesenchymal stromal cell treatment for refractory luminal Crohn's disease: results of a phase I study. Gut 59, 1662–1669. doi: 10.1136/gut.2010.215152
Duijvestein, M., Molendijk, I., Roelofs, H., Vos, A. C., Verhaar, A. P., Reinders, M. E., et al. (2011a). Mesenchymal stromal cell function is not affected by drugs used in the treatment of inflammatory bowel disease. Cytotherapy 13, 1066–1073. doi: 10.3109/14653249.2011.597379
Duijvestein, M., Wildenberg, M. E., Welling, M. M., Hennink, S., Molendijk, I., van Zuylen, V. L., et al. (2011b). Pretreatment with interferon-γ enhances the therapeutic activity of mesenchymal stromal cells in animal models of colitis. Stem Cells 29, 1549–1558. doi: 10.1002/stem.698
Fan, H., Zhao, G., Liu, L., Liu, F., Gong, W., Liu, X., et al. (2012). Pre-treatment with IL-1β enhances the efficacy of MSC transplantation in DSS-induced colitis. Cell. Mol. Immunol. 940, 473–481. doi: 10.1038/cmi.2012.40
Forbes, G. M., Sturm, M. J., Leong, R. W., Sparrow, M. P., Segarajasingam, D., Cummins, A. G., et al. (2014). A phase 2 study of allogeneic mesenchymal stromal cells for luminal Crohn's disease refractory to biologic therapy. Clin. Gastroenterol. Hepatol. 12, 64–71. doi: 10.1016/j.cgh.2013.06.021
François, M., Romieu-Mourez, R., Li, M., and Galipeau, J. (2012). Human MSC suppression correlates with cytokine induction of indoleamine 2,3-dioxygenase and bystander M2 macrophage differentiation. Mol. Ther. 20, 187–195. doi: 10.1038/mt.2011.189
Friedenstein, A. J. (1990). “Osteogenic stem cells in bone marrow,” in Bone and Mineral Research, eds J. N. M. Heersche and J. A. Kanis (Netherlands: Elsevier Science Publishers), 243–272.
Gao, F., Chiu, S., Motan, D., Zhang, Z., Chen, L., Ji, H.-L., et al. (2016). Mesenchymal stem cells and immunomodulation: current status and future prospects. Cell Death Dis. 7:e2062. doi: 10.1038/cddis.2015.327
Garg, S. K., Croft, A. M., and Bager, P. (2014). “Helminth therapy (worms) for induction of remission in inflammatory bowel disease,” in Cochrane Database of Systematic Reviews, ed S. K. Garg (Chichester: John Wiley & Sons, Ltd).
Glocker, E.-O., Kotlarz, D., Boztug, K., Michael Gertz, E., Schäffer, A. A., Noyan, F., et al. (2009). Inflammatory bowel disease and mutations affecting the interleukin-10 receptor. N. Engl. J. Med. 361, 2033–2045. doi: 10.1056/NEJMoa0907206
Gonzalez-Rey, E., Anderson, P., González, M. A., Rico, L., Büscher, D., and Delgado, M. (2009). Human adult stem cells derived from adipose tissue protect against experimental colitis and sepsis. Gut 58l, 929–939. doi: 10.1136/gut.2008.168534
Grimm, M. C., Pavli, P., Van De Pol, E., and Doe, W. F. (1995). Evidence for a CD14+ population of monocytes in inflammatory bowel disease mucosa-implications for pathogenesis. Clin. Exp. Immunol. 100, 291–297. doi: 10.1111/j.1365-2249.1995.tb03667.x
Hayashi, Y., Tsuji, S., Tsujii, M., Nishida, T., Ishii, S., Iijima, H., et al. (2008). Topical implantation of mesenchymal stem cells has beneficial effects on healing of experimental colitis in rats. J. Pharmacol. Exp. Ther. 326, 523–531. doi: 10.1124/jpet.108.137083
Henao Agudelo, J. S., Braga, T. T., Amano, M. T., Cenedeze, M. A., Cavinato, R. A., Peixoto-Santos, A. R., et al. (2017). Mesenchymal stromal cell-derived microvesicles regulate an internal pro-inflammatory program in activated macrophages. Front. Immunol. 8:881. doi: 10.3389/fimmu.2017.00881
Hill Charles, D., Mills, A. M., Kincaid, K., Alt, J. M., Mills, C. D., Heilman, M. J., et al. (2000). Paradigm M-1/M-2 Macrophages and the Th1/Th2 M-1/M-2 macrophages and the Th1/Th2 paradigm. J. Immunol. 164, 6166–6173. doi: 10.4049/jimmunol.164.12.6166
Honczarenko, M., Le, Y., Swierkowski, M., Ghiran, I., Glodek, A. M., and Silberstein, L. E. (2006). Human bone marrow stromal cells express a distinct set of biologically functional chemokine receptors. Stem Cells 24, 1030–1041. doi: 10.1634/stemcells.2005-0319
Hu, J., Zhao, G., Zhang, L., Qiao, C., Di, A., Gao, H., et al. (2016). Safety and therapeutic effect of mesenchymal stem cell infusion on moderate to severe ulcerative colitis. Exp. Ther. Med. 12, 2983–2989. doi: 10.3892/etm.2016.3724
Hunter, M. M., Wang, A., Parhar, K. S., Johnston, M. J. G., Van Rooijen, N., Beck, P. L., et al. (2010). In vitro-derived alternatively activated macrophages reduce colonic inflammation in mice. Gastroenterology 138, 1395–1405. doi: 10.1053/j.gastro.2009.12.041
Isidro, R. A., and Appleyard, C. B. (2016). Colonic macrophage polarization in homeostasis, inflammation, and cancer. Am. J. Physiol. Gastrointest. Liver Physiol. 311, G59–G73. doi: 10.1152/ajpgi.00123.2016
Italiani, P., and Boraschi, D. (2014). From Monocytes to M1/M2 Macrophages: phenotypical vs. functional differentiation. Front. Immunol. 5:514. doi: 10.3389/fimmu.2014.00514
Joeris, T., Müller-Luda, K., Agace, W., and Mowat, A. M. (2017). Diversity and functions of intestinal mononuclear phagocytes. Mucosal Immunol. 10, 845–864. doi: 10.1038/mi.2017.22
Kidd, S., Spaeth, E., Dembinski, J. L., Dietrich, M., Watson, K., Klopp, A., et al. (2009). Direct evidence of mesenchymal stem cell tropism for tumor and wounding microenvironments using in vivo bioluminescence imaging. Stem Cells 27, 2614–2623. doi: 10.1002/stem.187
Kudlik, G., Hegyi, B., Czibula, Á., Monostori, É., Buday, L., and Uher, F. (2016). Mesenchymal stem cells promote macrophage polarization toward M2b-like cells. Exp. Cell Res. 348, 36–45. doi: 10.1016/j.yexcr.2016.08.022
Lee, S. H., Starkey, P. M., and Gordon, S. (1985). Quantitative analysis of total macrophage content in adult mouse tissues. Immunochemical studies with monoclonal antibody F4/80. J. Exp. Med. 161, 475–489. doi: 10.1084/jem.161.3.475
Leung, G., Wang, A., Fernando, M., Phan, V. C., and McKay, D. M. (2013). Bone marrow-derived alternatively activated macrophages reduce colitis without promoting fibrosis: participation of IL-10. Am. J. Physiol. Gastrointest. Liver Physiol. 304, G781–G792. doi: 10.1152/ajpgi.00055.2013
Li, L., Liu, S., Xu, Y., Zhang, A., Jiang, J., Tan, W., et al. (2013). Human umbilical cord-derived mesenchymal stem cells downregulate inflammatory responses by shifting the Treg/Th17 profile in experimental colitis. Pharmacology 92, 257–264. doi: 10.1159/000354883
Li, Q., Wang, D., Hao, S., Han, X., Xia, Y., Li, X., et al. (2017). CD169 expressing macrophage, a key subset in mesenteric lymph nodes promotes mucosal inflammation in dextran sulfate sodium-induced colitis. Front. Immunol. 8:669. doi: 10.3389/fimmu.2017.00669
Liang, L., Dong, C., Chen, X., Fang, Z., Xu, J., Liu, M., et al. (2011). Human umbilical cord mesenchymal stem cells ameliorate mice trinitrobenzene sulfonic acid (TNBS)-induced colitis. Cell Transplant. 20, 1395–1408. doi: 10.3727/096368910X557245
Liu, W., Zhang, S., Gu, S., Sang, L., and Dai, C. (2015). Mesenchymal stem cells recruit macrophages to alleviate experimental colitis through TGFβ1. Cell. Physiol. Biochem. 35, 858–865. doi: 10.1159/000369743
Lo Sicco, C., Reverberi, D., Balbi, C., Ulivi, V., Principi, E., Pascucci, L., et al. (2017). Mesenchymal stem cell-derived extracellular vesicles as mediators of anti-inflammatory effects: endorsement of macrophage polarization. Stem Cells Transl. Med. 6, 1018–1028. doi: 10.1002/sctm.16-0363
Luk, F., de Witte, S. F., Korevaar, S. S., Roemeling-van Rhijn, M., Franquesa, M., Strini, T., et al. (2016). Inactivated mesenchymal stem cells maintain immunomodulatory capacity. Stem Cells Dev. 25, 1342–1354. doi: 10.1089/scd.2016.0068
Magatti, M., Vertua, E., De Munari, S., Caro, M., Caruso, M., Silini, A., et al. (2017). Human amnion favours tissue repair by inducing the M1-to-M2 switch and enhancing M2 macrophage features. J. Tissue Eng. Regen. 11, 2895–2911. doi: 10.1002/term.2193
Maggini, J., Mirkin, G., Bognanni, I., Holmberg, J., Piazzón, I. M., Nepomnaschy, I., et al. (2010). Mouse bone marrow-derived mesenchymal stromal cells turn activated macrophages into a regulatory-like profile. PLoS ONE 5:e9252. doi: 10.1371/journal.pone.0009252
Maheshwari, A., Kelly, D. R., Nicola, T., Ambalavanan, N., Jain, S. K., Murphy-Ullrich, J., et al. (2011). TGF-β 2 suppresses macrophage cytokine production and mucosal inflammatory responses in the developing intestine. Gastroenterology 140, 242–253. doi: 10.1053/j.gastro.2010.09.043
Manferdini, C., Paolella, F., Gabusi, E., Gambari, L., Piacentini, A., Filardo, G., et al. (2017). Adipose stromal cells mediated switching of the pro-inflammatory profile of M1-like macrophages is facilitated by PGE2: in vitro evaluation. Osteoarthr. Cartil. 25, 1161–1171. doi: 10.1016/j.joca.2017.01.011
Mantovani, A., Sica, A., Sozzani, S., Allavena, P., Vecchi, A., and Locati, M. (2004). The chemokine system in diverse forms of macrophage activation and polarization. Trends Immunol. 25, 677–686. doi: 10.1016/j.it.2004.09.015
Mao, F., Wu, Y., Tang, X., Kang, J., Zhang, B., Yan, Y., et al. (2017a). Exosomes derived from human umbilical cord mesenchymal stem cells relieve inflammatory bowel disease in mice. Biomed Res. Int. 2017:5356760. doi: 10.1155/2017/5356760
Mao, F., Wu, Y., Tang, X., Wang, J., Pan, Z., Zhang, P., et al. (2017b). Human umbilical cord mesenchymal stem cells alleviate inflammatory bowel disease through the regulation of 15-LOX-1 in macrophages. Biotechnol. Lett. 39, 929–938. doi: 10.1007/s10529-017-2315-4
Markovic, B. S., Nikolic, A., Gazdic, M., Nurkovic, J., Djordjevic, I., Arsenijevic, N., et al. (2015). Pharmacological inhibition of Gal-3 in mesenchymal stem cells enhances their capacity to promote alternative activation of macrophages in dextran sulphate sodium-induced colitis. Stem Cells Int. 2016:2640746. doi: 10.1155/2016/2640746
Martinez, F. O., and Gordon, S. (2014). The M1 and M2 paradigm of macrophage activation: time for reassessment. F1000Prime Rep. 6:13. doi: 10.12703/P6-13
Melief, S. M., Geutskens, S. B., Fibbe, W. E., and Roelofs, H. (2013a). Multipotent stromal cells skew monocytes towards an anti-inflammatory interleukin-10-producing phenotype by production of interleukin-6. Haematologica 98, 888–895. doi: 10.3324/haematol.2012.078055
Melief, S. M., Schrama, E., Brugman, M. H., Tiemessen, M. M., Hoogduijn, M. J., Fibbe, W. E., et al. (2013a). Multipotent stromal cells induce human regulatory T cells through a novel pathway involving skewing of monocytes toward anti-inflammatory macrophages. Stem Cells 31, 1980–1991. doi: 10.1002/stem.1432
Méndez-Ferrer, S., Michurina, T. V., Ferraro, F., Mazloom, A. R., Macarthur, B. D., Lira, S. A., et al. (2010). Mesenchymal and haematopoietic stem cells form a unique bone marrow niche. Nature 466, 829–834. doi: 10.1038/nature09262
Molendijk, I., Bonsing, B. A., Roelofs, H., Peeters, K. C., Wasser, M. N., Dijkstra, G., et al. (2015). Allogeneic bone marrow–derived mesenchymal stromal cells promote healing of refractory perianal fistulas in patients with Crohn's disease. Gastroenterology 149, 918.e6–927.e6. doi: 10.1053/j.gastro.2015.06.014
Murray, P. J. (2017). Macrophage polarization. Annu. Rev. Physiol. 79, 541–566. doi: 10.1146/annurev-physiol-022516-034339
Murray, P. J., Allen, J. E., Biswas, S. K., Fisher, E. A., Gilroy, D. W., Goerdt, S., et al. (2014). Macrophage activation and polarization: nomenclature and experimental guidelines. Immunity 41, 14–20. doi: 10.1016/j.immuni.2014.06.008
Nakajima, H., Uchida, K., Rodriguez Guerrero, A., Watanabe, S., Sugita, D., Takeura, N., et al. (2012). Transplantation of mesenchymal stem cells promotes an alternative pathway of macrophage activation and functional recovery after spinal cord injury. J. Neurotrauma 29, 1614–1625. doi: 10.1089/neu.2011.2109
Németh, K., Leelahavanichkul, A., Yuen, P. S. T., Mayer, B., Parmelee, A., Doi, K., et al. (2009). Bone marrow stromal cells attenuate sepsis via prostaglandin E2-dependent reprogramming of host macrophages to increase their interleukin-10 production. Nat. Med. 15, 42–49. doi: 10.1038/nm.1905
Onishi, R., Ohnishi, S., Higashi, R., Watari, M., Yamahara, K., Okubo, N., et al. (2015). Human amnion-derived mesenchymal stem cell transplantation ameliorates dextran sulfate sodium-induced severe colitis in rats. Cell Transplant. 24, 2601–2614. doi: 10.3727/096368915X687570
Owen, M., and Friedenstein, A. J. (1988). Stromal stem cells: marrow-derived osteogenic precursors. Ciba Found. Symp. 136, 42–60.
Panés, J., García-Olmo, D., Van Assche, G., Colombel, J. F., Reinisch, W., Baumgart, D. C., et al. (2016). Expanded allogeneic adipose-derived mesenchymal stem cells (Cx601) for complex perianal fistulas in Crohn's disease: a phase 3 randomised, double-blind controlled trial. Lancet 388, 1281–1290. doi: 10.1016/S0140-6736(16)31203-X
Parekkadan, B., Upadhyay, R., Dunham, J., Iwamoto, Y., Mizoguchi, E., Mizoguchi, A., et al. (2011). Bone marrow stromal cell transplants prevent experimental enterocolitis and require host CD11b+ Splenocytes. Gastroenterology 140, 966–975. doi: 10.1053/j.gastro.2010.10.013
Phinney, D. G., Di Giuseppe, M., Njah, J., Sala, E., Shiva, S., St Croix, C. M., et al. (2015). Mesenchymal stem cells use extracellular vesicles to outsource mitophagy and shuttle microRNAs. Nat. Commun. 6:8472. doi: 10.1038/ncomms9472
Popov, A., Driesen, J., Abdullah, Z., Wickenhauser, C., Beyer, M., Debey-Pascher, S., et al. (2008). Infection of myeloid dendritic cells with Listeria monocytogenes leads to the suppression of T cell function by multiple inhibitory mechanisms. J. Immunol. 181, 4976–4988. doi: 10.4049/jimmunol.181.7.4976
Porcheray, F., Viaud, S., Rimaniol, A.-C., Léone, C., Samah, B., Dereuddre-Bosquet, N., et al. (2005). Macrophage activation switching: an asset for the resolution of inflammation. Clin. Exp. Immunol. 142, 481–489. doi: 10.1111/j.1365-2249.2005.02934.x
Pull, S. L., Doherty, J. M., Mills, J. C., Gordon, J. I., Stappenbeck, T. S., and Olson, E. N. (2005). Activated macrophages are an adaptive element of the colonic epithelial progenitor niche necessary for regenerative responses to injury. Proc. Natl. Acad. Sci. U.S.A. 102, 99–104. doi: 10.1073/pnas.0405979102
Qian, B.-Z., and Pollard, J. W. (2010). Macrophage diversity enhances tumor progression and metastasis. Cell 141, 39–51. doi: 10.1016/j.cell.2010.03.014
Quarto, R., Mastrogiacomo, M., Cancedda, R., Kutepov, S. M., Mukhachev, V., Lavroukov, A., et al. (2001). Repair of large bone defects with the use of autologous bone marrow stromal cells. N. Engl. J. Med. 344, 385–386. doi: 10.1056/NEJM200102013440516
Romieu-Mourez, R., François, M., Boivin, M.-N., Stagg, J., and Galipeau, J. (2007). Regulation of MHC class II expression and antigen processing in murine and human mesenchymal stromal cells by IFN-gamma, TGF-beta, and cell density. J. Immunol. 179, 1549–1558. doi: 10.4049/jimmunol.179.3.1549
Rutgeerts, P., Sandborn, W. J., Feagan, B. G., Reinisch, W., Olson, A., Johanns, J., et al. (2005). Infliximab for induction and maintenance therapy for ulcerative colitis. N. Engl. J. Med. 353, 2462–2476. doi: 10.1056/NEJMoa050516
Sacchetti, B., Funari, A., Remoli, C., Giannicola, G., Kogler, G., Liedtke, S., et al. (2016). No identical “mesenchymal stem cells” at different times and sites: human committed progenitors of distinct origin and differentiation potential are incorporated as adventitial cells in microvessels. Stem Cell Rep. 6, 897–913. doi: 10.1016/j.stemcr.2016.05.011
Sala, E., Genua, M., Petti, L., Anselmo, A., Arena, V., Cibella, J., et al. (2015). Mesenchymal stem cells reduce colitis in mice via release of TSG6, independently of their localization to the intestine. Gastroenterology 149, 163.e20–176.e20. doi: 10.1053/j.gastro.2015.03.013
Selleri, S., Bifsha, P., Civini, S., Pacelli, C., Dieng, M. M., Lemieux, W., et al. (2016). Human mesenchymal stromal cell-secreted lactate induces M2-macrophage differentiation by metabolic reprogramming. Oncotarget 7, 30193–30210. doi: 10.18632/oncotarget.8623
Serena, C., Keiran, N., Madeira, A., Maymó-Masip, E., Ejarque, M., Terrón-Puig, M., et al. (2017). Crohn's disease disturbs the immune properties of human adipose-derived stem cells related to inflammasome activation. Stem Cell Rep. 9, 1109–1123. doi: 10.1016/j.stemcr.2017.07.014
Siegel, C. A. (2011). Review article: explaining risks of inflammatory bowel disease therapy to patients. Aliment. Pharmacol. Ther. 33, 23–32. doi: 10.1111/j.1365-2036.2010.04489.x
Smith, P., Mangan, N. E., Walsh, C. M., Fallon, R. E., McKenzie, A. N. J., van Rooijen, N., et al. (2007). Infection with a helminth parasite prevents experimental colitis via a macrophage-mediated mechanism. J. Immunol. 178, 4557–4566. doi: 10.4049/jimmunol.178.7.4557
Smith, T. D., Tse, M. J., Read, E. L., and Liu, W. F. (2016). Regulation of macrophage polarization and plasticity by complex activation signals. Integr. Biol. 8, 946–955. doi: 10.1039/C6IB00105J
Smythies, L. E., Sellers, M., Clements, R. H., Mosteller-Barnum, M., Meng, G., Benjamin, W. H., et al. (2005). Human intestinal macrophages display profound inflammatory anergy despite avid phagocytic and bacteriocidal activity. J. Clin. Invest. 115, 66–75. doi: 10.1172/JCI200519229
Song, J., Kang, H. J., Hong, J. S., Kim, C. J., Shim, J.-Y., Lee, C. W., et al. (2017). Umbilical cord-derived mesenchymal stem cell extracts reduce colitis in mice by re-polarizing intestinal macrophages. Sci. Rep. 7:9412. doi: 10.1038/s41598-017-09827-5
Song, W.-J., Li, Q., Ryu, M.-O., Ahn, J.-O., Bhang, D. H., Jung, Y. C., et al. (2017). TSG-6 secreted by human adipose tissue-derived mesenchymal stem cells ameliorates DSS-induced colitis by inducing M2 macrophage polarization in mice. Sci. Rep. 7:5187. doi: 10.1038/s41598-017-04766-7
Song, Y., Dou, H., Li, X., Zhao, X., Li, Y., Liu, D., et al. (2017). Exosomal miR-146a contributes to the enhanced therapeutic efficacy of interleukin-1β-primed mesenchymal stem cells against sepsis. Stem Cells 35, 1208–1221. doi: 10.1002/stem.2564
Stein, M., Keshav, S., Harris, N., and Gordon, S. (1992). Interleukin 4 potently enhances murine macrophage mannose receptor activity: a marker of alternative immunologic macrophage activation. J. Exp. Med. 176, 287–292. doi: 10.1084/jem.176.1.287
Takeyama, H., Mizushima, T., Uemura, M., Haraguchi, N., Nishimura, J., Hata, T., et al. (2017). Adipose-derived stem cells ameliorate experimental murine colitis via TSP-1-dependent activation of latent TGF-β. Dig. Dis. Sci. 62, 1963–1974. doi: 10.1007/s10620-017-4578-y
Tarique, A. A., Logan, J., Thomas, E., Holt, P. G., Sly, P. D., and Fantino, E. (2015). Phenotypic, functional, and plasticity features of classical and alternatively activated human macrophages. Am. J. Respir. Cell Mol. Biol. 53, 676–688. doi: 10.1165/rcmb.2015-0012OC
Thomas, A. C., and Mattila, J. T. (2014). “Of mice and men”: arginine metabolism in macrophages. Front. Immunol. 5:479. doi: 10.3389/fimmu.2014.00479
Tokuyama, H., Ueha, S., Kurachi, M., Matsushima, K., Moriyasu, F., Blumberg, R. S., et al. (2005). The simultaneous blockade of chemokine receptors CCR2, CCR5 and CXCR3 by a non-peptide chemokine receptor antagonist protects mice from dextran sodium sulfate-mediated colitis. Int. Immunol. 17, 1023–1034. doi: 10.1093/intimm/dxh284
Ueda, Y., Kayama, H., Jeon, S. G., Kusu, T., Isaka, Y., Rakugi, H., et al. (2010). Commensal microbiota induce LPS hyporesponsiveness in colonic macrophages via the production of IL-10. Int. Immunol. 22, 953–962. doi: 10.1093/intimm/dxq449
Ullman, T. A., and Itzkowitz, S. H. (2011). Intestinal inflammation and cancer. Gastroenterology 140, 1807.e1–1816.e1. doi: 10.1053/j.gastro.2011.01.057
Van Den Bossche, J., Baardman, J., Otto, N. A., Hoeksema, M. A., De Vos, A. F., De Winther Correspondence, M. P. J., et al. (2016). Mitochondrial dysfunction prevents repolarization of inflammatory macrophages. Cell Rep. 17, 684–696. doi: 10.1016/j.celrep.2016.09.008
Vasandan, A. B., Jahnavi, S., Shashank, C., Prasad, P., Kumar, A., and Prasanna, S. J. (2016). Human mesenchymal stem cells program macrophage plasticity by altering their metabolic status via a PGE 2-dependent mechanism. Sci. Rep. 6:38308. doi: 10.1038/srep38308
von Bahr, L., Sundberg, B., Lönnies, L., Sander, B., Karbach, H., Hägglund, H., et al. (2012). Long-term complications, immunologic effects, and role of passage for outcome in mesenchymal stromal cell therapy. Biol. Blood Marrow Transplant. 18, 557–564. doi: 10.1016/j.bbmt.2011.07.023
Vos, A. C., Wildenberg, M. E., Arijs, I., Duijvestein, M., Verhaar, A. P., de Hertogh, G., et al. (2012). Regulatory macrophages induced by infliximab are involved in healing in vivo and in vitro. Inflamm. Bowel Dis. 18, 401–408. doi: 10.1002/ibd.21818
Vos, A. C., Wildenberg, M. E., Duijvestein, M., Verhaar, A. P., van den Brink, G. R., and Hommes, D. W. (2011). Anti–tumor necrosis factor-α antibodies induce regulatory macrophages in an Fc region-dependent manner. Gastroenterology 140, 221.e3–230.e3. doi: 10.1053/j.gastro.2010.10.008
Wang, C., Chen, J., Sun, L., and Liu, Y. (2014). TGF-β signaling-dependent alleviation of dextran sulfate sodium-induced colitis by mesenchymal stem cell transplantation. Mol. Biol. Rep. 41, 4977–4983. doi: 10.1007/s11033-014-3364-6
Wei, H., Liu, X., Ouyang, C., Zhang, J., Chen, S., Lu, F., et al. (2017). Complications following stem cell therapy in inflammatory bowel disease. Curr. Stem Cell Res. Ther. 12, 471–475. doi: 10.2174/1574888X12666170315105556
Wei, H.-J., Zeng, R., Lu, J.-H., Lai, W.-F. T., Chen, W.-H., Liu, H.-Y., et al. (2015). Adipose-derived stem cells promote tumor initiation and accelerate tumor growth by interleukin-6 production. Oncotarget 6, 7713–7726. doi: 10.18632/oncotarget.3481
Wynn, T. A., Chawla, A., and Pollard, J. W. (2013). Macrophage biology in development, homeostasis and disease. Nature 496, 445–455. doi: 10.1038/nature12034
Xavier, R. J., and Podolsky, D. K. (2007). Unravelling the pathogenesis of inflammatory bowel disease. Nature 448, 427–434. doi: 10.1038/nature06005
Xue, J., Schmidt, S. V., Sander, J., Draffehn, A., Krebs, W., Quester, I., et al. (2014). Transcriptome-based network analysis reveals a spectrum model of human macrophage activation. Immunity 40, 274–288. doi: 10.1016/j.immuni.2014.01.006
Zhang, J., Lv, S., Liu, X., Song, B., and Shi, L. (2017). Umbilical cord mesenchymal stem cell treatment for Crohn's disease: a randomized controlled clinical trial. Gut Liver. 12, 73–78. doi: 10.5009/gnl17035
Zhang, Q.-Z., Su, W.-R., Shi, S.-H., Wilder-Smith, P., Xiang, A. P., Wong, A., et al. (2010). Human gingiva-derived mesenchymal stem cells elicit polarization of m2 macrophages and enhance cutaneous wound healing. Stem Cells 28, 1856–1868. doi: 10.1002/stem.503
Keywords: multipotent mesenchymal stromal cells, mesenchymal stem cells, inflammatory bowel disease, M1/M2 macrophage polarization, IL-10, PGE2
Citation: Hidalgo-Garcia L, Galvez J, Rodriguez-Cabezas ME and Anderson PO (2018) Can a Conversation Between Mesenchymal Stromal Cells and Macrophages Solve the Crisis in the Inflamed Intestine?. Front. Pharmacol. 9:179. doi: 10.3389/fphar.2018.00179
Received: 14 December 2017; Accepted: 16 February 2018;
Published: 06 March 2018.
Edited by:
Gaetano Cairo, Università degli Studi di Milano, ItalyReviewed by:
Manfred Nairz, Innsbruck Medical University, AustriaAlessandro Poggi, Ospedale Policlinico San Martino, Italy
Copyright © 2018 Hidalgo-Garcia, Galvez, Rodriguez-Cabezas and Anderson. This is an open-access article distributed under the terms of the Creative Commons Attribution License (CC BY). The use, distribution or reproduction in other forums is permitted, provided the original author(s) and the copyright owner are credited and that the original publication in this journal is cited, in accordance with accepted academic practice. No use, distribution or reproduction is permitted which does not comply with these terms.
*Correspondence: Per O. Anderson, per.anderson@genyo.es
†These authors have contributed equally to this work.